Physicists at MIT capture the first images of individual atoms freely interacting in space, revealing correlations among particles that were previously predicted but never directly observed.
Physicists have captured the first images of individual atoms freely interacting in space.
The pictures reveal correlations among the ‘free-range‘ particles that until now were predicted but never directly observed.
These findings, appearing today in the journal Physical Review Letters, will help scientists visualize never-before-seen quantum phenomena in real space.
Atoms are the smallest units of matter, consisting of a nucleus surrounded by electrons.
They are the fundamental building blocks of everything around us, from the air we breathe to the stars in the universe.
Atoms are incredibly small, with sizes measured in picometers.
There are 118 known elements, each with its unique atomic number and mass.
Understanding atoms is crucial for various fields, including chemistry, physics, and materials science.
The Power of Atom-Resolved Microscopy
Bosons and fermions are two fundamental categories of particles in the Standard Model of particle physics.
Bosons are particles with integer spin, such as photons (spin 1) and gluons (spin 1), which mediate forces between other particles.
Fermions, on the other hand, have half-integer spin and include electrons, quarks, and neutrinos.
The distinction between bosons and fermions is crucial in understanding particle interactions and behavior.
Researchers developed a technique called atom-resolved microscopy, which allows a cloud of atoms to move and interact freely before freezing their motion using a lattice of light.
This technique enables scientists to directly observe the behavior of individual atoms interacting in free space.
The team applied this technique to visualize clouds of different types of atoms, snapping a number of imaging firsts.
Bosons and Fermions: Unlocking Quantum Secrets
The researchers directly observed interactions among both bosons and fermions.
Photons are an example of a boson, while electrons are a type of fermion.
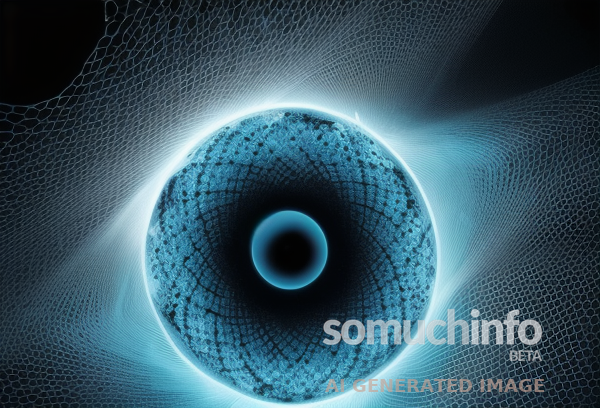
Atoms can be bosons or fermions, depending on their total spin, which is determined by whether the total number of their protons, neutrons, and electrons is even or odd.
The study revealed that bosons exhibit ‘bunching,’ having an increased probability to be near each other due to their ability to share one and the same quantum mechanical wave.
A Breakthrough in Understanding Quantum Phenomena
The MIT team’s achievement provides a significant step forward in understanding quantum phenomena, which have long been challenging to observe directly.
The study’s findings demonstrate that scientists can visualize the behavior of individual atoms interacting in free space, shedding light on previously theoretical concepts.
This breakthrough has far-reaching implications for our understanding of superconductivity and other quantum phenomena.
Atoms are the fundamental units of matter, and their interactions determine the properties and behavior of substances.
Atoms interact through electromagnetic forces, which act between charged particles such as protons and electrons.
These forces can be either attractive or repulsive, depending on the charge of the particles involved.
The strength of atomic interactions depends on factors like distance, charge, and spin.
Understanding atom-atom interactions is crucial for explaining chemical reactions, phase transitions, and material properties.
Future Directions
The team plans to apply their imaging technique to visualize more exotic and less understood phenomena, such as ‘quantum Hall physics‘ — situations when interacting electrons display novel correlated behaviors in the presence of a magnetic field.
With this technology, scientists can verify whether theoretical models are accurate representations of real-world phenomena, advancing our understanding of quantum mechanics.
Supporting Research
This work was supported by various funding agencies, including the National Science Foundation and the Air Force Office of Scientific Research.
The research was conducted at the MIT-Harvard Center for Ultracold Atoms, a collaborative effort between two world-renowned institutions.